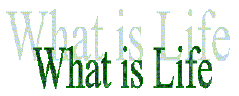
Pentose Phosphate Pathway and Glycogen*
Metabolism
Glucose-6-phosphate
is a key metabolite at the crossroad of four different pathways.
First, under aerobic conditions and at low ATP (high ADP) concentrations,
glucose-6-P will rapidly be oxidized to CO2 via pyruvate
and acetyl-CoA. Second, when in need for biosynthetic activity,
such as cell division with a great need for new nucleic acids, some
of the glucose-6-P oxidation proceeds via ribulose-5-P in the pentose
phosphate pathway. Third, phosphatases provide free glucose in
liver that rapidly equilibrates across the hepatic cell membrane,
a mechanism by which the liver controls blood glucose levels. Fourth,
to store extra glucose for emergencies independent of sugar levels
in the blood as glycogen in liver and muscle cells (carbohydrate
loading).
Pentose Phosphate Pathway
(KEGG
pathway MAP00030)
The pentose phosphate pathway (or cycle) converts glucose-6-phosphate
to reducing equivalents (NADPH) and biosynthetic precursors (ribose)
for the reductive biosynthesis of lipids and nucleic acids. The
pathway can be modulated according to different metabolic requirements
such as:
Pathway
Mode |
Result |
Net
Reaction |
Oxidative
branch |
Reducing
power and pentoses |
6
glucose-6-P + 12 NADP+ Þ
6 ribulose-5-P + 6 CO2 + 12 NADPH |
Non-oxidative
branch |
Glycolytic
intermediates |
6
ribulose-5-P Þ 5 glucose-6-P
+ Pi |
Both
branches (cyclic) |
Reducing
power |
1
glucose-6-P + 12 NADP+ Þ
6 CO2 + 12 NADPH + Pi |
The first step of the oxidative branch is catalyzed by
Glucose-6-phosphate
1-dehydrogenase (E.C. 1.1.1.49) and competes with
phosphoglucoisomerase
(E.C. 5.3.1.9) for glycolysis and phosphoglucomutase
(E.C. 5.4.2.2) for glycogen synthesis for its substrate. This linear
branch involves two dehydrogenases, one which decarboxylates a C6
lactone intermediate to a C5 sugar. It generates the nucleotide
precursor ribose-5-phosphate which is a component of important coenzymes
ATP, CoA, NAD(P)+, FAD, and genetic material RNA, and DNA.
The second mode, or non-oxidative branch, of the pentose
phosphate cycle includes several interconversion steps of C7, C4,
and C3 ketoses
and aldoses
regenerating glucose-6-phosphate, fructose-6-phosphate, and glyceraldehyde-3-phosphate,
all three being metabolites of glycolysis/gluconeogenesis.
This complex recycling of ribulose-5-P back to glucose-6-P is necessary
for the complete anaerobic oxidation of one molecule of glucose
to carbon dioxide and water and 12 molecules of NADPH (the last
mode shown above).
All reactions of the non-oxidative branch are fully reversible,
unlike the dehydrogenase reactions of the oxidative branch of the
pentose phosphate pathway. The decarboxylation makes the oxidative
reaction irreversible. Only plants and photosynthetic microorganisms
have the enzymes necessary to reverse this pathway (Calvin cycle)
using closely related, but not identical set of proteins.
Glycogen
metabolism
(KEGG
Pathway MAP00500)
Glucose cannot accumulate in large numbers inside cells because
an accumulation of charged glucose-6-P would have a strong osmotic
effect essentially causing cells to swell. Instead, glucose is stored
in form of the non-charged, water free glucose polymer glycogen.
After a heavy intake of carbohydrates, one tenth of the liver mass
may exist as glycogen. Within the first 12 to 24 hours after food
withdrawal most of the bodies carbohydrate needs are met by this
internal storage. After this period the body switches to gluconeogenesis
to meet the carbohydrate needs, primarily fueled by amino acid metabolism,
i.e. protein degradation. The normal glycogen content of liver varies
between 2 and 8%.
Glycogenesis is the synthesis of glycogen glucose-6-P
is isomerized to glucose-1-P by phosphoglucomutase
and subsequently activated by UTP as an high energy biosynthetic
precursor UDP-glucose.
UTP + glucose-1-P Þ UDP-glucose
+ PPi
The highly energy compound UDP-glucose is used to transfer a glucose
unit to branches of glycogen. This reaction is catalyzed by glycogen
synthetase ( E.C. 2.4.1.11). The nucleotide of choice for
glucose activation for glycogen synthesis in E.coli is ATP
forming ADP-glucose from glucose-1-P.
Glycogenolysis or glycogen degradation uses phosphorylase
(E.C. 2.4.1.1) to remove glucose units from by phosphorylation (instead
of hydrolysis) to form free glucose-1-P.
Glycogen synthesis and degradation are co-regulated through
intracellular (allosteric) as well as extracellular (hormonal) signals.
The intracellular regulation is mediated by the levels of ATP and
AMP, while the hormonal control operates through posttranslational
enzyme phosphorylation and dephosphorylation. The hormones glucagon
and epinephrine stimulate glycogen degradation through enzyme phosphorylation
inhibiting its synthesis. Insulin is an antagonist to glucagon and
epinephrine stimulating glycogen synthesis via dephosphorylation
of glycogen synthase (now active) and phosphorylase (now inactive).
Thus, with a single switch, glycogen synthesis can be activated
(inhibited) while its degradation is inhibited (activated).
In the absence of hormonal activation, cytoplasmic AMP activates
phosphorylase b. ATP competes with AMP inhibits phosphorylase
b. The phosphorylation of phosphorylase b by kinase,
however, converts it to the fully active phosphorylase a
independent of ATP and AMP levels. Thus hormonal control overrides
a cellular (or local) control mechanism.
Go to table of contents
|