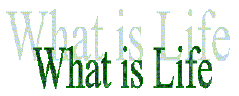
Nitric oxide
Overview
(KEGG
pathway MAP00330 for arginine and proline metabolism)
The small, free radical molecule nitric
oxide (NO; N=O) has been identified as a major signal transduction
molecule in vertebrates (animals). NO is derived from arginine in
two steps catalyzed by nitric
oxide synthase (NOS; EC 1.14.13.39). NOS catalyzes the net
reaction:
L-Arginine + n NADPH + m O2 = Citrulline + Nitric oxide + n NADP+
with the intermediate N-(omega)-Hydroxyarginine (C05933). The catalytic
activity of nitric oxide synthase is related to the monooxygenase
activity of cytochrome P450. This catalytic relationship
becomes apparent when comparing the gene structure of both enzymes.
Nitric oxide synthase is the larger of the two enzymes. Its C-terminal
domain is identical to the smaller Cyt P450 protein.
There are three forms of nitric oxide synthase - a neuronal type
called nNOS, an epithelial type called eNOS, and an inducible form
called iNOS. The latter is only expressed under certain conditions
like immune system regulation by cytokines or pathological induction
in the presence of endotoxins (bacterial lipopolysaccharide) and
cytotoxins (which affect cytokine secretion). NO production is a
stress response and can lead to either tissue injury because of
its radical chemistry, or be cytoprotective, protecting cells from
damage by destroying pathogenic microorganisms first. For
example, stomach ulcers have lately been associated with a bacterial
infection. Helicobacter pylori (H.pylori) causes ulcers and
gastric cancers. Nitric oxide and particularly its superoxide derivative
peroxynitrite cause DNA damage in the bacteria.
Free
radicals as antimicrobials: |
ONOO
induces DNA damage through chemical modifications (mutations)
while NO inhibits ribonucleotide reductase. Both DNA damage
and reductase inhibition keep the cell in a state of energy
costly nucleotide synthesis and repair mode. This leads eventually
to cell death by energy depletion of bacterial cells.
|
Such defense mechanism, however, have their draw backs. Inducible
NOS, which is expressed as an emergency mechanism to suppress tumor
growth in gastric epithelia, breast tissue, and the brain,
is linked to septic shock. Bacterial endotoxins (e.g. from H.pylori
or E.coli infections) induce the iNOS gene, which in turn
produces high levels of NO damaging pathogenic DNA and inhibiting
respiration (inhibits metabolic energy production needed for cell
division). The free radicals, however, cannot discriminate pathogenic
DNA from host DNA and overstimulation of iNOS therefore induces
cell and tissue damage, sometimes leading to a fatal development
(septic shock) in the course of bacterial infections. This is a
well known situation in hospitals affecting patients with an already
suppressed immune system.
The neuronal and epithelial NOS isoforms are constitutively expressed
and regulated by calcium concentration via calmodulin interaction.
The calcium-calmodulin complex stabilizes the homodimer. Each monomer
contains a reductase and oxygenase subunit containing FAD, NADPH
& FMN or heme & tetrahydrobiopterine (B4H) cofactors, respectively.
B4H is essential for NOS dimer formation. (H4biopterine is an important
cofactor of aromatic amino acid hydroxylases.) Nitric oxide synthase
is a membrane bound protein, anchored to the cytoplasmic side of
endoplasmatic reticulum, Golgi, or plasma membrane by myristoylation
or palmitoylation. The lipid anchored NOS are preferentially found
in cholesterol and glycolipid rich membrane domains. The compartmentalization
of NOS appears to be crucial for its functionality by providing
local NO levels.
NOS regulation
The activity of eNOS and nNOS is controlled by tetrahydrobiopterin
and Ca/CaM availability because these two cofactors are needed for
the proper dimer formation of an active synthetase. The dependence
on calmodulin has been used as a model to explain the role of glutamate
in neurotoxicity in the central nervous system. Neurotoxicity is
a mechanism of glutamate induced neuronal cell death. The immediate
effect of glutamate on neurons is its role in activating glutamate
receptor, namely to pharmacological subtypes known as NMDA Receptors
(NMDA is a methylated derivative of aspartate). Glutamate receptors
are selective for calcium ions. Thus, prolonged activation of glutamate
receptors stimulates eNOS via Ca/CaM complex binding to the synthetase.
The formation of NO is implicated in cell death as described above:
DNA damage, suppressed mitochondrial respiration, leading to energy
depletion. Neurons are particularly sensitive to impaired mitochondrial
ATP synthesis capacity, because neurons depend almost exclusively
on the oxidative degradation of glucose and ketone bodies. The formed
ATP is used by ion selective pumps to maintain the proper ion gradients
for action potential generation and neurotransmitter release of
presynaptic membranes.
NO can only be synthesized, however, if the amino acid arginine
is available. Neuronal NOS critically depends on this substrate,
which is mainly synthesized in adjacent glial cells and is transported
into neurons. Arginine uptake into neurons is controlled by non-NMDA
glutamate receptors. This became evident when these receptors were
blocked by arginine-uptake inhibitors such as L-lysine which functions
as antagonist of these glutamate receptors. The physiological role
of nNOS in mechanisms such as long term potentiation has been shown
to involve retrograde transport (diffusion) of NO synthesized in
post synaptic neurons across the synaptic cleft into synapses, where
they stimulated guanyl cyclase.
Nitric oxide and free
radical biochemistry
Nitric oxide is a free radical molecule and its major effect is
the activation of cytoplasmic, soluble guanyl cyclase
(sGC; EC 4.6.1.2). This enzyme catalyzes the cyclization of GTP
to cGMP + PPi. Cyclic GMP is a signaling molecule (similar to cAMP)
by virtue of activating protein kinases. .
Nitric oxide binds to the heme group of cyclase. Other protein
targets are metallo enzymes, where NO binds to Fe-S clusters. Aconitase
is inactivated by NO, as is complex IV, the cytochrome oxidase in
the inner membrane of mitochondria. Thus NO as an inhibitory effect
on oxidative phosphorylation by blocking the electron transport
chain and controlling the levels of citrate in the Krebs cycle essentially
blocking the oxidative degradation of acetyl-CoA.
NO is a short lived chemical transmitter, which is freely diffusible
across membranes. The molecule possesses a small dipole moment because
of the similar electronegativity of oxygen and nitrogen, making
it essentially hydrophobic. Its reactivity is due to the unpaired
electron in the outer valence orbital of its oxygen constituent.
NO is almost unreactive as free radical as compared to other oxygen
radicals. Indeed, NO decays within seconds after its synthesis if
left unbound in solution because it reacts with either molecular
oxygen or superoxide.
NO strongly interacts with molecular oxygen to form dinitrotrioxide
(N2O3), or with superoxide O2-. to form peroxynitrite
(ONOO-). NO also binds to sulfhydryl groups (SH) and
unsaturated fatty acids. The reaction with superoxide can be diminished
by superoxide dismutase (SOD) which removes O2-. to form
hydrogen peroxide (H2O2). NO can be 'stored' by covalent interaction
to glutathione to form S-nitroso-glutathion. Both H2O2 and S-nitrosoglutathion
can have a stimulatory effect on guanine cyclase. Superoxide dismutase
thereby prevents the loss of nitric oxide to peroxynitrite forming
hydrogen peroxide instead and increasing the cyclase stimulatory
capacity of the cell. See glutathione metabolism for details MAP00480.
NO can potentially be regenerated from ONOO- in two
steps; a first reduction of peroxynitrite by cytochrome C oxidase
to nitrite (NO2), followed by a reduction of nitrite to NO by the
enzyme nitrate reductase. The latter enzyme exists in two
isoforms, a mitochondrial type and an endoplasmatic reticulum resident
protein. Both receive their electrons needed for nitrite reduction
to NO from either NADH or NADPH, and interact with flavoproteins
(FAD prosthetic groups) and cytochromes (cytochrome c oxidase in
mitochondrial membrane; cytochrome P450 in ER membrane).
Cytochrome C oxidase and nitrite reductase therefore reduce the
concentration of highly reactive, secondary metabolites, and potentially
contribute to NO signaling. The latter has only been shown in plant
cells, where nitrate reductase reaction appears to be a considerable
contributor to this signaling molecule.
Peroxynitrite, hydrogen peroxide, and dinitrotrioxide all have
been linked to cell death (apoptosis = programmed cell death) through
protein nitration and increased mutagenesis. The latter is a consequence
of DNA stand breakage and guanine nitration. For example, acute
neural toxicity is linked to the overproduction of peroxynitrite,
which inhibits respiratory enzymes and also damages DNA by covalent
bond formation to DNA and removal of bases. Inhibitors of nitric
oxide synthase and antioxidants are known to have neuroprotective
properties because the limit the formation of highly reactive nitrogen
containing radicals.
Antioxidants
The free radical chemistry in cells can be prevented or at least
diminished by adding antioxidants or free radical scavengers, molecules
which have a high affinity and strongly react with these free radicals.
Antioxidants are either hydrophilic or hydrophobic. Hydrophilic
antioxidants include glutathione peroxidase, Fe(II) chelators like
the proteins ceruloplasmin and transferrin, and hydroxylated aromatic
molecules like uric acid or ascorbate (vitamin C). Hydrophobic antioxidants
include flavin-nucleotide or carotene containing proteins and vitamin
E.
Melatonin too is a major physiological antioxidant (and hormone)
by directly reacting with hydroxyl and peroxyl radicals, or by stimulating
the expression of superoxide dismutase, glutathione peroxidase,
or glutathione reductase. Melatonin has also been reported to inhibit
nitric oxide synthetase.
Physiological role of
NO as neurotransmitter
In epithelial cells, NO causes vascular dilatation by controlling
smooth muscle contractility. In the central nervous system it affects
synaptic transmission stimulating learning and memory capacity.
Glutamate is produced and released by a synapse and activates the
NMDA receptor subtype of glutamate receptors. This leads to an influx
of calcium ions which in turn bind to calmodulin, activating the
neuronal NOS. NOS synthesizes NO depending on the availability of
L-arginine, which is mainly supplied from extra-neuronal sites (mainly
glial cells). NO not only activates the postsynaptic guanyl cyclases,
but can diffuse across the synaptic cleft back into the synapse
that originally released the glutamate. This retrograde transport
of NO is thought to reinforce the capability of glutaminergic signaling.
Such a prolonged reinforcement of synaptic stimulatory activity
is known as long term potentiation and is implicated as a
possible molecular mechanism promoting long term memory and learning.
In blood plasma NO induces platelet aggregation, an important factor
in wound healing and blood coagulation. It has been shown that hemoglobin
is a major transport vehicle for NO in blood.
Go to table of contents
|