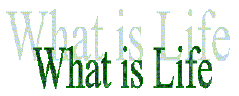
Oxidative Phosphorylation
Overview
(KEGG
pathway MAP00190)
The reductive power generated by the citric acid cycle in form
of NADH/H+ can be utilized by the inner mitochondrial
membrane to generate ATP. Reducing equivalents harnessed in the
Krebs cycle, fatty acid oxidation, and pyruvate dehydrogenase activity
are transferred to membrane bound electron transport chain. Four
classes of electron transport complexes segregate electron and protons
promoting proton pumping across the inner membrane using molecular
oxygen as a final electron acceptor ('sink') reducing oxygen to
water. The proton gradient across the inner membrane drives the
ATP synthase to regenerate ATP from ADP and inorganic phosphate.
This process is also known as respiration.
The Mitochondrion
Mitochondria are small organelles with possible common origin to
bacteria (endosymbiotic theory). They contain a double membrane
system. The outer membrane is relatively permeable to small metabolites.
This is due to the presence of a porin channel type VDAC (voltage
dependent anion channel) with a high copy number
(thousands of channel in a single mitochondrion) with preferences
for negatively charged ions (nucleotides, phosphorylated compounds).
Large molecules like proteins, however, are not able to cross the
outer membrane. The size limit for permeable molecules is about
1,000 Dalton. The mitochondrial inner membrane functions as electrical
insulator and capacitor and is impermeable for ions and small hydrophilic
metabolites. This membrane maintains the ion (proton) gradient essential
for ATP synthesis. The matrix side is negatively charged relative
to the outside (inter-membrane space; connected to cytoplasm through
VDAC) because of the proton gradient (high out; low in) generated
during the electron transport chain reaction. This membrane contains
many of the substrate specific transport systems like citrate, glycerol,
and malate shuttles, but also the ATP-ADP exchange protein. It is
the latter exchange transport that controls the speed of citric
acid cycle, oxidative phosphorylation and thus all precursor pathways
like glycolysis, protein degradation and fatty acid oxidation.
Electron carriers in
oxidative phosphorylation
Electron carriers along the electron transfer chain come in two
forms; they bind reducing equivalents or electrons only. Combining
both types within a chain forces the separation of protons from
electrons. While the electrons stay within the membrane, protons
are captured and released from and into the surrounding compartments.
This process is unidirectional and always picks up protons in the
matrix compartments and releases them in the inter-membrane space
thus creating a proton gradient which stores energy in form of electrochemical
potential.
The first category of reducing equivalent acceptors riboflavin
(vitamin B2) and nicotinamine (vitamin B3 or nicacin). Riboflavin
is part of FAD and FMN and is analogous to that of nicotinamide
in NAD(P) by accepting hydrogens and electrons into its heterocyclic
ring structures. Two important differences to NAD(P), however, exist.
First, flavin-adenine
dinucleotide (FAD; or FADH2, the reduced flavin-
adenine- dinucleotide; C01352) and flavin
mono nucleotide (FMN oxidized C00061; reduced
FMN C01847) are prosthetic groups (covalent link to enzyme)
and do not carry reducing equivalents by diffusion. Second, the
reduction of FAD and FMN by NADH is not reversible. Flavoproteins
participate at several points where electrons are first funneled
into the respiratory chain:
NADH + H+ + Enz-FMN Þ
NAD+ + Enz-FMNH2
The reduction potential of this reaction D
E0' = 0.3V corresponding to about -46kcal/mol of standard
free energy change, enough for the synthesis of two to three mols
of ATP per mol of oxidized NADH.
The second category of reducing equivalent carriers is the benzoquinone
(oxidized) conatining compound coenzyme
Q. Like flavoproteins they accept two hydrogens along with two
electrons upon reduction of the hydroquinone
ring structure to ubiquinole or CoQH2. The quinone-hydroquinone
redox couple serves as a diffusible transport system within the
inner membrane of mitochondria coupling the electron flux with a
proton flux across the dielectric barrier by providing a non-charged
carrier system. Quinones serve as a collector molecule of reducing
equivalents (e-/H+) from NADH and succinate
donors.
Redox systems of the respiratory chain that only bind electrons
are the Fe-S complexes and heme groups (Fe-proto-porphyrin ring;
protoheme
C00032). The irons of the heme groups serve as redox partners
by reversibly changing their redox state between the reduced Fe(II)
and the oxidized Fe(III) form. Synthesis of heme can be found in
KEGG
as MAP00860 (Porphyrin and chlorophyll metabolism).
Table of individual protein
complex systems in oxidative phosphorylation
NADH
dehydrogenase (complex I) |
ENTRY EC 1.6.5.3: Complex
I is the first coupling site in the mitochondrial membrane
meaning that the redox reaction is coupled to a proton pumping
activity across the membrane. It is the energy stored in the
electrochemical proton gradient that is used for ATP synthesis
by the H+-ATPase or complex V. Complex I is the entry
point for NADH reducing power and involves FMN, Fe-S complexes,
and ubiquinone. |
Succinate
dehydrogenase (complex II) |
ENTRY EC
1.3.5.1 is the mitochondrial succinate dehydrogenase and
unlike complex I does not directly contribute to proton pumping.
The reducing equivalents from succinate are transferred by FAD
to ubiquinone. |
Cytochrome-c
reductase (complex III) |
Complex III or cytochrome
bc1 complex (EC 1.10.2.2) transfers electrons from quinones
to cytochrome c, a small peripheral membrane protein (cytochrome
c; C00524) in the inter-membrane space. This complex contributes
to proton pumping in a mechanism known as Q-cycle. A similar
mechanism is also hypothesized to work in complex I. |
Cytochrome-c
oxidase (complex IV) |
Cytochrome c transfer single electrons from complex
III to complex IV, or cytochrome-c
oxidase (EC 1.9.3.1). As can be seen from the
KEGG entry for cytochrom c oxidase, the human complex IV contains
up to eight subunits. For some subunits, cell type specific
homologues exists such as COX7A1 in muscle and COX7A2 in liver.
Cytochrome oxidase catalyzes a cyclic reaction in which the
electrons extracted from metabolites are finally transferred
to molecular oxygen in the presence of 4 protons to form 2 molecules
of H2O. This is a carefully controlled mechanism because the
intermediate oxygen radicals are highly reactive and damaging
to the cell. Oxygen is tightly bound to an iron-copper containing
coenzyme. |
H+-ATPase
(complex V) |
The mitochondrial ATP
synthase (EC 3.6.1.34) is a multi-subunit protein complex
that couples a proton channel (F0 portion, integral
membrane protein complex) with an ATP synthesizing unit (F1
portion, soluble mitochondrial matrix component) and is a member
of the so called F-type ATPases. |
For an on-line demonstration of the Q-cycle go to Science
Media and choose the 'Biochemical Interactions' thumbnail.
Go to table of contents
|