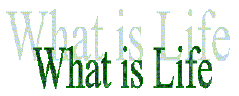
Citric Acid Cycle (Krebs Cycle)
Overview
(KEGG
pathway MAP00020)
The citric acid cycle, discovered in 1937 in animal
tissue, is the central metabolic pathway for all aerobic processes.
The cycle provides the complete oxidation of C2 units (acetyl-CoA)
derived from fats, carbohydrates and lipids into carbon dioxide
and water capturing the released energy as reductive power in
the form of NADH and FADH2. The cycle also provides
metabolic intermediates for biosynthetic purposes.
The enzymes and intermediates of the citric acid cycle are found
inside the mitochondrial matrix, where the reductive power of NADH
and FADH2 can directly be fed (in form of electrons) into the electron
transport chain of the oxidative phosphorylation process in the
inner membrane of this organelle. This electron flow is coupled
to proton flow which is temporarily stored as an electrochemical
gradient (proton motif force or PMF) which in turn is harvested
by the ATP synthase to produce chemical energy.
The substrate of the cycle is an activated C2 unit - acetate in
the form of Acetyl-Coenzyme A. It is derived from glycolysis by
a decarboxylating dehydrogenase activity of pyruvate
dehydrogenase. This mitochondrial enzyme is organized in
three major protein complexes forming a particle larger than a ribosome.
Complex |
E1
|
E2
|
E3
|
Name |
Pyruvate
dehydrogenase |
Dihydrolipoamide
S-acetyltransferasee |
Dihydrolipoamide
dehydrogenase |
Subunits |
24
subunits (EC 1.2.1.51 & 1.2.4.1) |
24
subunits (EC 2.3.1.12) |
12
subunits (EC 1.8.1.4) |
Coenzyme |
Thiamin
pyrophosphate (TPP)
|
Lipoamide,
Coenzyme A |
FAD,
NAD |
Reaction |
Oxidative
decarboxylation of pyruvate to form actyl-TPP; release of carbon
dioxide renders reaction irreversible |
Acetyl
transfer via lipoamide to diffusible CoA |
FAD
oxidizes lipoamide; FAD oxidized by NAD which is reduced to
NADH/H+ |
The net reaction of pyruvate dehydrogenase is:
pyruvate + CoA + NAD+ = acetyl-CoA + CO2 + NADH + H+
The energy contained is extracted by a cyclic set of reaction producing
reducing equivalents and one molecule of GTP through a complete
oxidation to two carbon dioxide molecules. The C2 unit in the form
of an activated acetyl molecule, acetyl-CoA, is combined
in a first step with the C4 unit oxaloacetate to form a C6 unit,
citrate. Citrate is then oxidized and decarboxylated via a C5 intermediate
(alpha-keto-glutarate) to oxaloacetate (C4). Starting from oxaloacetate,
the full cycle adds two carbon units from acetate (CH3-COOH) and
yields two carbon units as 2 CO2, 3 NADH, 1 GTP, and
1 FADH2 in form of chemical energy and 1 oxaloacetate.
The cycle is non-reversible meaning that it cannot use CO2
for acetate formation. The following is a step-by-step description
of the regeneration of oxaloacetate from citrate:
The cycle yields the following intermediates, some of which can
be used as biosynthetic precursors.
The oxidation steps include lipoamide
dehydrogenase (EC 1.8.1.4). This enzyme is a component (subunit)
of both multienzymes pyruvate dehydrogenase complex (E.Cs. 1.2.4.1
+ 1.8.1.4 + 2.3.1.12) and (E.Cs. 1.2.4.2 + 1.8.1.4).
In fact, the usage of enzyme complex subunits in different enzymes
with different substrate specificity but identical reaction mechanism
(i.e., use of coenzymes), is commonly found in biological systems.
Biosynthetic function
and control of citric acid cycle
As for glycolysis and gluconeogenesis, the citric acid cycle is
regulated by the energy needs of the cell, mainly the rate of oxidative
phosphorylation to replenish cellular ATP. Therefore, ATP and NADH
exert a negative feed back control on citrate synthase (the
first step in the cycle that feeds acetyl-CoA into it) and iso-citrate
dehydrogenase, the first step in generating reductive power.
This two allosteric regulations completely shut down the cycle when
ATP synthesis is no longer needed and NADH starts to accumulate.
Because both the Krebs cycle and oxidative phosphorylation are localized
in the mitochondrial matrix, there is an immediate diffusion-controlled
feed back mechanism to the key enzymes in both pathways. In addition,
this allosteric control shuts down the cycle under anaerobic conditions,
where NADH and FADH2 can no longer be oxidized by the electron transport
chain components (complexes I & II).
A second regulatory mechanism is the feed back control of citrate
on glycolysis and fatty acid synthesis. High citrate concentrations
are indicative of high acetyl-CoA levels slowing down glycolysis
while accelerating fatty acid synthesis reduces acetyl-CoA levels.
Excess oxaloacetate not needed in the Krebs cycle (e.g. anaerobic
conditions) will then be funneled into gluconeogenesis. The absolute
concentration of oxaloacetate is the major focal point in metabolic
coordination between energy household and biosynthetic demands by
linking the metabolisms of carbohydrates, lipids, and amino acids
together.
Citric acid cycle in
E.coli and plants
The citric acid cycle is highly conserved in all organisms. There
are metabolic differences, however, under different circumstances.
In E.coli the citric acid cycle switches to a branched, non-cyclic
mode under anaerobic conditions. The cycle is 'broken' at the alpha-ketoglutarate
dehydrogenase step and takes a reverse path to succinyl-CoA starting
from oxaloacetate via malate/aspartate, fumarate, and succinate.
The branched citric acid 'cycle' mode provides biosynthetic precursors
succinyl-CoA and alpha-ketoglutarate. Oxaloacetate itself
is used up in this reaction and needs to be replenished from the
combination of two acetate units produced in the complete oxidation
of pyruvate. This is performed by the glyoxylate cycle in
bacteria (and plants; in the organelles called glyoxysomes). In
this cycle, isocitrate is split by isocitrate lyase into succinate
and glyoxylate. The latter can be linked with acetyl-CoA to form
malate and CoA in a reaction catalyzed by malate synthase.
Acetyl-CoA is formed from ATP and acetate by a phosphoryl group
transfer to form acetyl-phosphate and ADP. The activated acetate-P
can be moved to a CoA to form acetyl-CoA and inorganic phosphate.
Go to table of contents
|