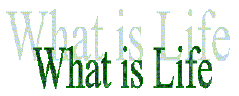
Phospholipids and Membrane Biogenesis
Cell Membranes
Membranes are barriers for hydrophilic molecules and ions because
of the hydrophobic core of the phospholipid bilayer. A membrane
or phospholipid bilayer is a two-dimensional, spherical particle
separating an inside compartment from an outside compartment. In
addition to lipids, a membrane contains membrane proteins
that control the transport of hydrophilic and charged, small and
large molecules into and out of the cell and intracellular organelles.
The importance of the lipid bilayer membrane is its ability to function
as an electrical capacitor. This enables charge separation
and thus the storage of electro-chemical energy in form of ion gradients.
One example is the proton motif force (pmf or proton gradient) discussed
in sections on oxidative phosphorylation and photosynthesis. Membrane
proteins that serve as conductors are used by the cell to extract
small quanta of this energy for synthesis or signaling mechanisms.
Two examples are ATP synthesis and action potentials, respectively.
Phospholipids
(KEGG pathways MAP00561)
The main structural component of biological membranes are the phospholipids.
The biosynthesis phospholipids depends on the syntesis of diacylglycerol.
Diacylglycerol in liver comes from phosphatidic
acid (C00416; 1,2-Diacyl-sn-glycerol 3-phosphate). Phosphatidic
acid can be derived from glycerol
(C00116) by phosporylating it at the expense of ATP to glycerol-3-phosphate
(C00093). The enzyme catalyzing the reaction is glycerol
kinase (EC 2.7.1.30). Glycerol-3-P can also be obtained
from reduction of the glycolytic intermediate dihydroxyacetonephosphate
(DAHP; C00111) by glycerol-3-P
dehydrogenase (EC 1.1.99.5, 1.1.1.94, 1.1.1.8 isoforms).
Glycerol-3-phosphate is then twice esterified using acyl-CoA precursors
as fatty acid donors to phosphatidic acid. The first acylation to
1-Acyl-sn-glycerol
3-phosphate (C00681) is catalyzed by Glycerol-3-phosphate
O-acyltransferase (EC 2.3.1.15) and the second fatty acylation
is catalyzed by 1-Acylglycerol-3-phosphate
O-acyltransferase (EC 2.3.1.51) to 1,2-Diacyl-sn-glycerol
3-phosphate (systematic name) or phosphatidic acid (common name).
The first acylation of glycerol-phosphate takes place at either
the endoplasmatic reticulum membrane or the mitochondrial membrane.
The latter reaction is significant in liver, an organ with a large
numbers of mitochondria. The product of this acylation is lysophosphatidic
acid. Note that at this time this and all following metabolites
are membrane components, including all enzymes catalyzing the synthesis
of membrane lipids.
Phosphatidic acid is a minor component of cell membranes but an
essential intermediate for both fat synthesis and phospholipid synthesis.
Fat or triglyceride
(C00422) are synthesized from phosphatidic acid by dephosphorylation
to 1,2-diglyceride
(C00641) by Phosphatidate
phosphatase (EC 3.1.3.4). A third acyl-CoA unit is then
used to esterify the third carbon position in the glycerol backbone
by the enzyme Diglyceride
acyltransferase (EC 2.3.1.20) to form triglycerides.
1,2-diglyceride is a precursor for both phosphatidylcholine
(C00157, lecithin) and phosphatidylethanolamine
(C00350) by combining the diacylglycerol with an activated choline
(ethanolamine) group using the nucleoside CTP to form CDP-choline
(C00307) and CDP-ethanolamine
(C00570), respectively. Choline is activated by phosphorylation
using ATP to phosphorylcholine and phosphorylcholine in turn is
formed to CDP-choline by CTP releasing pyrophosphate as byproduct.
These committed reactions are analogous to the activation of glucose-1-P
to UDP-glucose for glycogen synthesis. Phosphatidylethanolamine
can also serve as precursor for phosphatidylcholine by transfer
of three activated choline groups In addition, phosphatidylserine
is derived from phosphatidylethanolamine by exchanging the ethanolamine
headgroup for serine.
Two minor phospholipids are phosphatidylglycerol (PG) and phosphatidylinositol
(PI). They are derived from phosphatidic acid instead of 1,2-diglyceride.
Phosphatidic acid is activated by activation using CTP to form the
high energy compound CDP-diglyceride
(C00269). The cytosine unit is replaced by either inositol to form
phosphatidylinositol (PI) and CMP, or glycerol-3-phosphate to form
phosphatidylglycerol (PG) and CMP and Pi. Both PG and
PI are negatively charged phospholipids because of the single negative
charge contributed by the phosphate ester linkage.
Fatty acid elongation
and desaturation (oxidation)
Phospholipids can be distinguished not only by their headgroup
chemical properties, but also their fatty acid composition. The
latter varies in terms of acyl chain length and degree of desaturation,
or oxidation. The cytoplasmic fatty
acid synthetase complex only forms palmitoyl-CoA as end product.
Longer fatty acids, predominantly C18 and the monounsaturated derivatives
of C16 and C18 are commonly found in biological membranes.
In eukaryotes the elongation of of fatty acids beyond C16 length
is performed by enzyme systems of the endoplasmatic reticulum
membrane, with the active sites facing the cytoplasmic side
of this membrane. The substrate for fatty acid elongation and desaturation
is the acyl-CoA form, although some cells or organisms may use directly
phospholipids. The diverse acyl-CoA moieties are subsequently acylated
to glycerol-3-P and lysophosphatidic acid as described above.
To elongate fatty acyl chains (C16 or longer) by C2 units at their
carboxyl end, malonyl-CoA is used as donor for both saturated
and unsaturated fatty acids. The elongation process starting from
C16:0 can be repeated to produce C18:0, C20:0, C22:0, and C24:0
fatty acids. These latter fatty acids are important for sphingomyelin
formation in brain.
Oxidation (desaturation) requires NADPH/H+ and molecular
oxygen in a reaction involving a three-enzyme complex: NADPH-cytochrome
b5 reductase (FAD coenzyme), cytochrome b5 (heme), and
D9-desaturase (non-heme iron).
The latter oxidizes stearoyl-CoA to oleoyl-CoA. The reducing equivalent
from NADPH/H+ together with the electron/hydrogen pairs
gained from cis C=C bond formation are used to reduce one
molecular oxygen to two water molecules. The net oxidation of the
saturated C18:0 fatty acid (stearoyl) to a monounsaturated state
(C18:1; oleoyl) is given as:
Stearoyl-CoA + NADPH + H+ + O2 Þ
Oleoyl-CoA + NADP+ + 2H2O
A single cis C=C bond is introduced in this reaction resides
between carbon 9 and carbon 10. The bond is referred to as cisD9.
As an example, look at Phosphatidylcholine
desaturase (EC 1.3.1.35). Its substrates are linoleate and
oleate.
Note, there is no pathway included in KEGG on phospholipid elongation
or desaturation. Desaturases of fatty acids do not produce chemical
energy and their mechanism is fundamentally different from the dehydrogenase
activities of mitochondrial enzymes of the Krebs cycle and beta-oxidation
pathways. Here, reducing power is invested to support the
oxidation of fatty acids. These desaturases are also distinct from
so called monooxygenases (cytochrome P450 or mixed function oxygenases)
which will be discussed in the chapter on sterol
synthesis. As their name suggests, the lipid substrate is oxygenated
(epoxide, hydroxyl) in the process.
Mammals do not have enzymes to oxidize fatty acids carbon bonds
beyond the C9-C10 position. Thus in mammals there exist desaturases
for positions 4, 5, and 6, but not for 12 or 15. Most polyunsaturated
fatty acids in human metabolism depend on the two essential
fatty acids. Linoleate
(C18:2 D9,D12;
KEGG C01595) and linolenate (C18:3 D9,D12,D15)
are part of dietary lipids and are precursors in mammalian cells
for the synthesis of eicosanoids that function as signaling
molecules in cell-cell communication, development, and inflammation
(prostaglandin
and leukotrienes).
Assembly of lipids into
membranes
The process of lipid insertion into membranes can be split into
three major parts. First, the initial incorporation of newly synthesized
lipid into an acceptor membrane. Second, the generation of the proper,
i.e., asymmetric, transmembrane distribution of lipids. Third, maintenance
of the proper lipid composition in the various target membranes
for which de novo synthesized lipids have been produced.
The mechanism of the first step appears obvious based on the above
discussed ER membrane bound enzyme systems responsible for the synthesis
of phosphatidic acid, phospholipids, and triglycerides. The substrates
and products of these enzyme mechanisms are located in the outer
leaflet of the ER membrane. The second mechanism requires the redistribution
of phospholipids from the outer to the inner leaflet (or monolayer)
of the ER membrane. This process is not random, for all cell membranes
are found to contain an asymmetric composition of lipid components
in the inner and outer monolayers. Specialized membrane proteins
called flippases transport the hydrophilic headgroup portion
of these amphipathic molecules across the hydrophobic fatty acid
core of the ER membrane.
Little is known about the specifics of these transport and sorting
mechanisms. One membrane protein in ER membranes is an ATP driven
'pump' that shuttles phospholipids from the cytoplasmic monolayer,
the site of synthesis, to the lumenal side, which corresponds to
the extra-cellular side of the plasma membrane. An alternative mechanism
has been suggested for the synthesis of PC, which is found preferentially
on the cell surface. In this model, a transmembrane methylase
uses PE on the cytoplasmic side of the membrane as substrate and
sequentially methylates the headgroup to choline forming PC. In
the process, the phospholipid is transported across the membrane
with the choline headgroup facing the lumenal side. De novo
synthesized membranes are transported via a vesicle mechanism to
the Golgi complex and from there to different organelles and the
plasma membrane. The orientation of the two monolayers, the cytoplasmic
surface and the lumenal side, are strictly maintained.
Lipoprotein particles
The major site of lipid synthesis is the endoplasmatic reticulum
membrane of the liver. Lipids therefore have to be transported to
other membranes in the same cell and from liver cells to other tissues.
Inside cells, membrane vesicles are transported between various
organelles and the plasma membrane. Outside cells, lipids are transported
in association with serum proteins, albumins and lipoprotein particles.
Lipoprotein particles are heterogeneous complexes with various
blood plasma protein fractions carrying triglycerides, phospholipids
and cholesterol. Together, they compose the blood lipid levels which
measures about 500mg/100ml and exhibits wide fluctuations depending
on dietary sources. Lipoprotein particles are distinguished on the
basis of their physical density. Chylomicrons show the lowest density
of all lipoproteins having the highest lipid to protein ratio of
all. Chylomicrons are composed mostly of fats (from diet absorption)
with a thin protein layer that keeps the particle soluble. The very
low density lipoproteins (VLDL) have a high lipid content but contain
more cholesterol and phospholipids making them more dense than chylomicrons.
Low density lipoproteins (LDL) have the highest ratio of cholesterol,
while high density lipoproteins (HDL) have the highest phospholipid
content. The very high density lipoproteins (VHDL) contain mostly
albumin and carry mostly free fatty acids.
With the exception of albumin, all protein components of lipoprotein
particles are classified as globins. Alpha globins are the major
component of HDLs and beta globins the major component of LDLs.
Each of the lipoprotein classes play a distinct role in lipid transport.
VLDLs carry fats from liver to other tissues (dietary fats come
from mucosal cells via chylomicrons). The major target tissue is
adipose tissue. After unloading its triglycerides the VLDL is converted
to an LDL particle. LDL carries the bulk of plasma cholesterol.
The targets are peripheral tissues and the cholesterol exists in
an esterified form. The origin of these cholesterol esters is liver
cholesterol which upon its secretion from hepatocytes is acylated
by the plasma enzyme lecithin-cholesterol acyl transferase that
transfers an unsaturated fatty acid from position 2 of phosphatidylcholine
to cholesterol. The left-over phospholipid - lysophosphatidylcholine-
can be re-acylated in red blood cells to PC. The exchange of lipids
from lipoproteins occurs directly with the cell membranes of the
target cells. The unloaded lipoprotein is now a VHDL containing
mostly protein fractions (albumin bound fatty acids) and can be
recycled by the liver.
Atherosclerosis
Atherosclerotic lesions consist of cholesterol deposits in the
intimal lining of the arteries. Eventually, the lumen may become
occluded to the extent that the blood flow is impaired. This causes
restricted oxygen supply to peripheral organs like the brain, back
pressure on the major arteries that can lead to high blood pressure
and congestive heart failure. Blood platelets and debris can accumulate
at the plaque deposits further narrowing the circulation. Chronic
high ratios of LDL ('bad cholesterol') over HDL particles is thought
to be the major cause of these cholesterol deposits.
Go to table of contents
|